Imagine this: It’s a chilly evening in Bern, Switzerland, in 1905. A young patent clerk named Albert Einstein sits alone in his modest apartment, scribbling notes under the dim glow of a lamp. The room is cluttered with papers, calculations scrawled in the margins.
He pauses, lost in thought, staring out the window. Outside, life carries on — people stroll past, unaware that the man upstairs is about to change the world. In that moment, Einstein isn’t thinking about fame or revolutionizing science. He’s just chasing an idea — one that refuses to leave his mind.
Little does he know, the paper he’s about to complete will shatter centuries of classical physics and unlock the mysteries of light, atoms, and time itself.
Bern, 1905: A 26-year-old patent clerk, Albert Einstein scribbles ideas by lamplight that will upend centuries of science. In the span of a single year, Albert Einstein published four papers that shattered the foundations of classical physics.
One introduces light “quanta” – a radical notion that light comes in particle-like packets – to solve a puzzling effect no one could explain. Another confirms that invisible atoms are real by decoding the jittery dance of pollen in water.
A third redefines space and time itself. It shows that time can stretch and shrink and that mass and energy are two sides of the same coin. This “Annus Mirabilis” (miracle year) of 1905 forever changed our perception of reality, lighting the fuse for the quantum revolution, E = mc², and modern physics technologies we rely on every day.
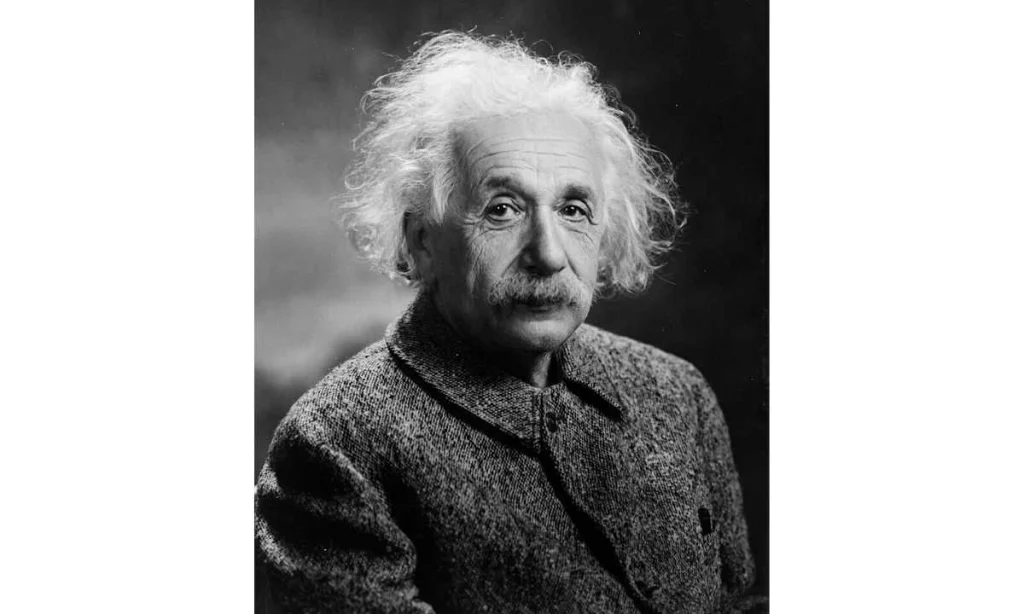
The Mysteries That Stumped Classical Physics
By the early 20th century, physics was at a crossroads. Experiments were revealing mysteries that Newton’s laws and Maxwell’s waves couldn’t solve:
- The Photoelectric Puzzle: Shining light on metal can knock out electrons (the photoelectric effect), but with weird twists. Brighter light (higher intensity) ejects more electrons without making them any faster. It only light above a certain frequency (color) can even kick out electrons at all.
- Classical wave theory predicted the opposite – more intense light should impart more energy – yet reality disagreed.
- It was a glaring inconsistency: why did ultraviolet light eject electrons where red light failed, even if the red light was insanely bright? The wave theory of light was unable to explain this effect.
- The Reality of Atoms: Many scientists still weren’t fully convinced that atoms and molecules were real physical entities in 1905. A phenomenon called Brownian motion. It is the random jitter of microscopic pollen in water – has been observed for decades. However, no one could definitively explain it. Were invisible molecules battering the pollen grains? If so, it would be direct evidence of atomic reality, ending the skepticism that matter might be continuous. It was an open problem: how to prove the existence of atoms that no microscope could see.
- Conflicting Visions of Space and Time: Classical mechanics (Newton) assumed time was absolute and the laws of physics the same for all observers moving at a constant speed. But Maxwell’s electromagnetism had shown light is an electromagnetic wave with a fixed speed (~300,000 km/s) – fixed relative to what? If you raced alongside a beam of light, classical logic said you should see the light wave frozen in place – an absurd scenario no experiment supported.
- Physicists postulated an invisible “ether” filling space as the medium for light waves, but the idea was failing experimental tests. A new framework was needed to reconcile Maxwell’s light with Newton’s mechanics and remove the growing contradictions about how time and speed worked at high velocities.
Each of these puzzles – a chink in the armor of classical physics – begged for a solution. In 1905, one little-known physicist cracked them all.
The Miraculous Year: Einstein’s Journey of Discovery
In 1905, Albert Einstein was a patent examiner in Bern, Switzerland – “a venerable federal pen-pusher,” as he joked, far from academia
Yet in his free time this young man unleashed a blitz of breakthroughs. Einstein later recalled that his time in Bern was “the most creative of my life.” The explosion of insight that would puzzle science historians for decades
How did he do it?
Einstein met regularly with friends in a self-styled “Olympia Academy,” debating physics and philosophy in Bern’s cafés
Fueled by intellectual freedom (and perhaps the monotony of examining patent applications), he attacked physics’ big problems as a lone detective on a mission. In March 1905, he sent off his first paper – and it was a bombshell. In it, Einstein dared to propose a new picture of light to solve the photoelectric conundrum.
He imagined light as a stream of tiny packets of energy – later dubbed photons – rather than a purely continuous wave. Building on Max Planck’s quantum idea (from 1900) that energy comes in discrete units.
Einstein extended it to light itself: each particle of light carries an energy E = h·f (Planck’s constant h times the light frequency f). This bold hypothesis implied that whether electrons get ejected from a metal depends on the energy of individual light quanta, not the light’s overall intensity.
A low-frequency red photon is like a gentle tap. No single one has the punch to knock an electron out of its atom. A higher-frequency violet or UV photon is like a swift kick. Even one can liberate an electron if it carries enough energy. Thus, increasing light intensity just adds more “taps” (more photons). It knocks out more electrons but not making each electron any more energetic.
Only a higher-frequency (higher-energy) photon can give an electron a faster kick, explaining why ultraviolet light could succeed where red light failed.
Einstein’s “light quanta” theory was radically out of step with entrenched wave theory – truly a eureka moment. Even he felt it: he later remarked that this idea was the most revolutionary of his 1905 works, because it pushed physics into the new quantum realm
It explained the photoelectric effect beautifully. The study even predicted a precise linear relationship between the light frequency and the kinetic energy of ejected electrons. This could be tested – and indeed, by 1916 Robert Millikan’s meticulous experiments confirmed Einstein’s photoelectric equation, verifying that energy is proportional to frequency
The once-skeptical Millikan (who had set out to disprove Einstein) was astonished to measure Planck’s constant from photoelectron energies, proving that Einstein’s constant h was the same Planck had found in blackbody radiation
The last doubts evaporated. In 1921 Einstein was awarded the Nobel Prize in Physics specifically for “his discovery of the law of the photoelectric effect.” It highlighted how the old wave theory had failed and Einstein’s particle insight prevailed
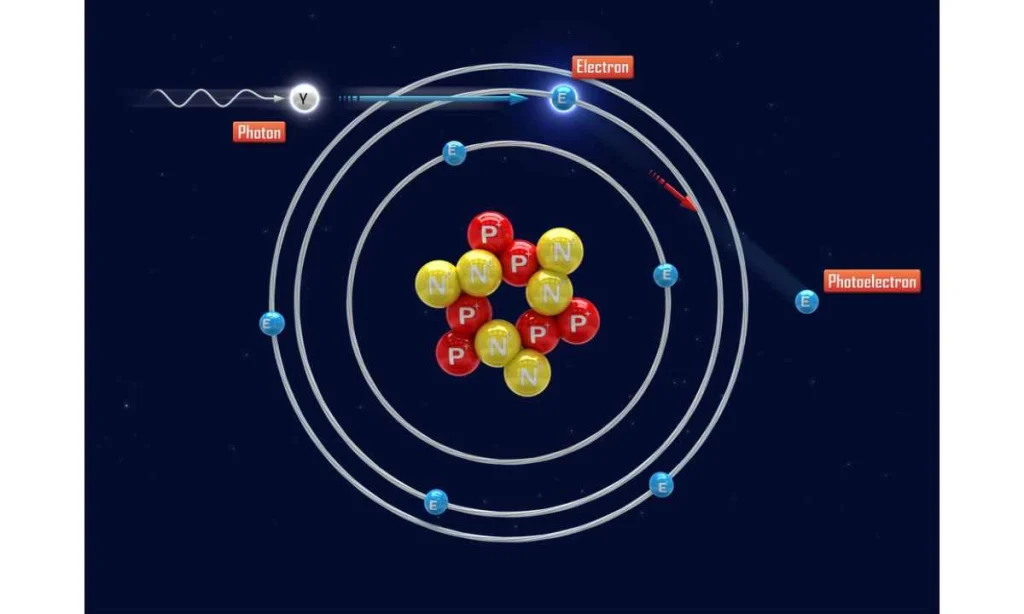
Higher-frequency light (e.g. UV) can liberate electrons (blue E), while lower-frequency light (e.g. red) cannot, regardless of intensity.
That spring and summer of 1905, Einstein didn’t stop at light quanta. He swiftly turned to the Brownian motion problem – effectively providing a mathematical model that treated pollen jostled by invisible molecules. Using statistical physics, he showed that if atoms were real and in constant random motion, they would impart a jitter to suspended particles that matched the erratic, jittery paths observed under the microscope
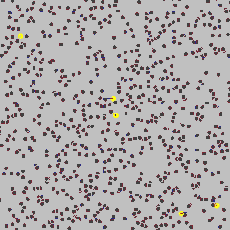
He derived formulas for how far a particle would wander over time, linking the microscopic collisions to observable diffusion. This work provided a concrete test of the atomic hypothesis by measuring Brownian motion, one could calculate the size of atoms or the number of molecules in a given volume (Avogadro’s number). It was the evidence atom skeptics needed. A few years later, in 1908, physicist Jean Perrin meticulously confirmed Einstein’s predictions, measuring the displacement of particles and nailing down Avogadro’s number
Perrin’s experiments ended the long-standing skepticism about the existence of atoms and molecules as actual physical entities
In Einstein’s hands, the unseen world of molecules became perceptible; reality was made of atoms, and now everyone believed it.
Finally, in June 1905, Einstein completed a paper titled “On the Electrodynamics of Moving Bodies,” introducing what we now call the Special Theory of Relativity. Here, the hero was not a new particle or medium.
But a simple rethinking of space and time. Einstein realized that Maxwell’s laws of electromagnetism – and the constant speed of light – could only make sense if Newton’s absolute time was abandoned.
He boldly postulated two principles: (1) The laws of physics are the same for all inertial observers (no preferred frame of reference, “no ether wind” needed), and (2) the speed of light is constant for all observers, no matter how fast they move.
These two statements, though separately reasonable, together force a startling conclusion: measurements of space and time are relative. Einstein worked out the consequences with elegant thought experiments: moving clocks tick slower, moving objects contract in length along the direction of motion, and simultaneity is not universal – two events that seem simultaneous in one frame may occur at different times in another.
He showed that time can dilate and lengths can contract such that everyone still measures light traveling at 300,000 km/s.
The old absolute notions of time and space dissolved into a new space-time continuum. By September 1905, Einstein wrote a follow-up note deriving the iconic equation emerging from special relativity: E = m·c².
Mass and energy, he demonstrated, are deeply connected – a small amount of mass can convert to an enormous amount of energy. This was no thought experiment curiosity; it was a glimpse at how stars shine and, decades later, how humans would tap nuclear energy.
Incredibly, all four of these papers were authored by an “unknown” young man outside academia. As physicist Arnold Sommerfeld later quipped, each paper “was worth a Nobel Prize” on its own.
Einstein had essentially become three different pioneers in one year: a quantum theorist, a pioneering atomist, and a relativist.
“It is… pretty unusual that three such spectacular contributions would come from an unknown patent clerk all in one yea. that’s why physicists celebrate Einstein’s 1905”
noted physicist David Gross.
The world gradually awoke to Einstein’s genius. By 1908 he gained a professorship, and within a decade his name was synonymous with the scientific revolution.
Reality Redefined: From Light Quanta to the Quantum Revolution
Einstein’s 1905 papers didn’t just solve academic puzzles – they revolutionized physics and launched entire fields. His notion of light quanta in particular cracked open the door to the bizarre new world of quantum mechanics.
Understanding the photoelectric effect was “fundamentally significant in the development of modern physics” because it forced physicists to accept that light (and later electrons and other matter) has a dual wave–particle nature. Einstein’s work showed that sometimes light behaves as a particle (photon) with discrete energy
This concept was soon extended by Bohr, Schrödinger, Heisenberg, and others into a full quantum theory of matter by the 1920s. In a real sense, Einstein’s light-quanta hypothesis helped launch the quantum revolution, paving the way for all the quantum physics of the 20th century.
His relativity, meanwhile, demolished the rigid Newtonian framework of absolute space and time. After 1905, space and time became malleable, intertwined dimensions. This set the stage for Einstein’s own General Relativity in 1915 (gravity as curved spacetime). However, even special relativity alone was transformative. It unified energy and matter (E = mc²) and accurately described particles moving near light-speed.
Without it, modern particle physics and cosmology would be impossible. And Einstein’s proof of atoms via Brownian motion anchored the statistical mechanics of gases and liquids. It gave confidence in using atomic theory to understand chemistry, thermodynamics, and materials.
The impact on human knowledge was seismic.
“Changed the course of physics forever, and radically altered human conceptions of reality.”
As the directors of the Kavli Institute noted on the centennial, 1905’s trio of landmark papers.
The idea that time can run differently for different observers, or that light can be both wave and particle, was as mind-bending to the early 1900s public as it is awe-inspiring to us today. Einstein had essentially shown that nature’s laws are sometimes counter-intuitive, yet deeper and more beautiful than previously imagined.
From Theory to Technology: Modern Applications of Einstein’s 1905 Discoveries
What’s truly astonishing is how these once-esoteric ideas have become the backbone of today’s technology and industry. Einstein in 1905 could scarcely have imagined the devices and systems that his theories would eventually enable. Here are some of the real-world technologies that trace directly back to Einstein’s “miracle year” insights:
- Solar Panels and Photocells: Every solar power panel and digital camera sensor today operates on the photoelectric principle Einstein explained. When photons hit a semiconductor, they eject electrons to create a current – just as in the metal experiments Einstein described.
- Understanding this light-to-electricity conversion paved the way for solar cells that harvest sunlight and photo-detectors that underpin imaging. The “electric eye” sensors that open automatic doors, light meters in photography, and Xerox photocopying all sprang from the photoelectric effect. Thanks to Einstein’s work, we can turn sunlight into electricity on rooftops around the world.
- Lasers and Fiber Optics: The laser – a staple of modern communication, medicine, and electronics – owes its existence to Einstein’s quantum ideas. In 1917, Einstein built on his 1905 light quantum theory to predict stimulated emission, the process that makes lasers possible.
- He showed that an excited atom could be induced to emit an identical photon, multiplying light of a single frequencyndupress.ndu.edu. This concept lay dormant for decades until engineers in the 1950s demonstrated the first maser and laser. Today, lasers scan barcodes, perform eye surgery, carry signals through fiber-optic cables, and read Blu-ray discs.
- It all goes back to Einstein’s realization that atoms could emit coherent light on demand – “Einstein’s discovery provided the basis for the development of lasers.” Every time you play a CD or use a laser pointer, you’re tapping into the quantum physics that Einstein helped establish.
- Quantum Computing and Photonics: The latest frontier in computing harnesses the very quantum principles that Einstein’s 1905 work helped introduce. Quantum computers use qubits that can exist in superpositions of states, leveraging the wave-particle duality and entanglement – phenomena born from early quantum theory. It’s fitting that Einstein, who confirmed the wave-particle duality of light with the photoelectric effect, indirectly seeded the ideas that power quantum information science.
- Photons (light quanta) are leading candidates for qubits in quantum communication, and understanding their behavior is rooted in Einstein’s century-old insights. Even Einstein’s famous skepticism of quantum mechanics (his “spooky action at a distance” quip about entanglementimd.org) spurred debate that led to today’s quantum technologies.
- Modern quantum cryptography, for instance, often uses single photons – particles of light as Einstein conceived – to securely transmit encryption keys. In short, our emerging quantum computing era stands on the shoulders of Einstein’s early quantum leaps.
- Precision Timekeeping – Atomic Clocks and GPS: If you used GPS navigation today, you implicitly trusted Einstein’s relativity. GPS satellites orbiting Earth carry ultra-precise atomic clocks, and to give you an accurate position, those clocks must account for both Special and General Relativity. Because satellites move fast (14,000 km/h) and sit high above Earth’s gravity, their clocks tick slightly faster than ones on the ground. Engineers apply Einstein’s time dilation corrections – a net adjustment of about 38 microseconds per day – to synchronize GPS time.
- Without this, GPS would drift by kilometers. The fact that your smartphone can pinpoint your location is direct evidence that time and space behave exactly as Einstein predicted. In laboratories, atomic clocks now measure time so precisely that physicists have observed relativistic effects over mere millimeters of elevation – literally seeing time run faster a few floors up in a building.
- Einstein’s relativity isn’t just abstract: it’s ticking away in every satnav unit and confirming itself with each nanosecond. The atomic clock, too, relies on quantum theory – the fixed energy levels of atoms (another legacy of early quantum physics) – to keep time with incredible accuracy. These clocks enable global telecommunications, internet timestamping, and financial transaction timing. In sum, from Google Maps to stock trades, Einstein’s 1905 ideas keep the world on schedule.
The IBM Q quantum computer (white cylindrical cryostats shown) is a direct descendant of Einstein’s quantum revolution. By affirming that light and matter have discrete quantum states
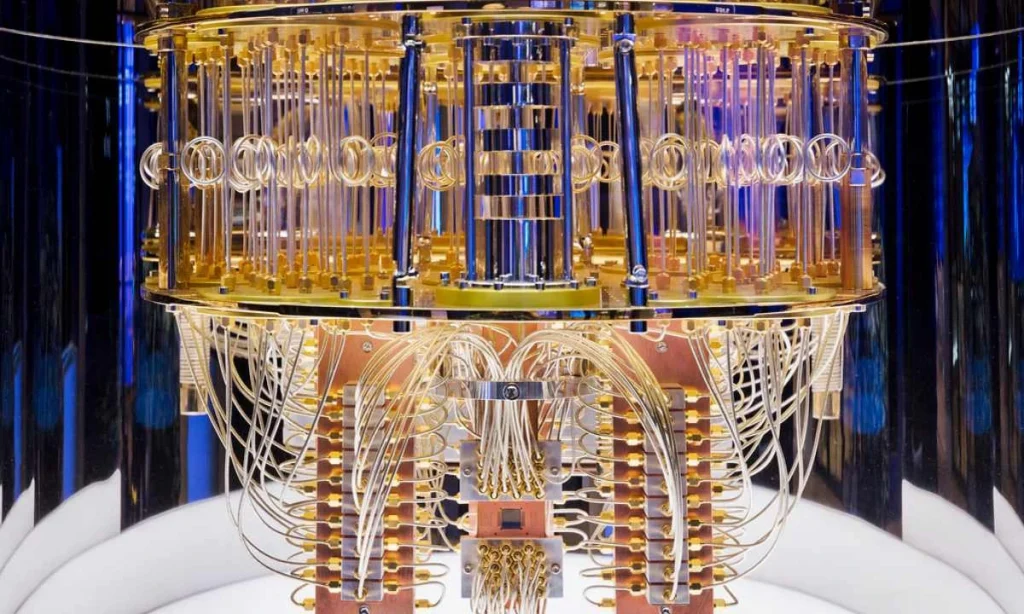
- Energy = mc² and Nuclear Power: While not yet applied in 1905, Einstein’s mass–energy equivalence soon proved its power. In the 1930s and 40s, scientists realized that E = mc² underpinned nuclear reactions. The sunshine that warms Earth is produced by mass converting to energy in the Sun’s core, exactly as Einstein’s formula dictates.
- Human civilization harnessed this in nuclear reactors and (destructively) in atomic bombs. Whenever we generate nuclear power or medical isotopes, we are using the fact that a tiny amount of mass can release tremendous energy – Einstein’s 1905 insight made manifest.
- The equation also drives our understanding of particle physics: creating new particles in accelerators “costs” energy per mc², and annihilating matter releases energy. Thus, from the macro (power plants, star energy) to the micro (particle-antiparticle creation), Einstein’s relativity has transformed technology and science.
This list could go on – satellite communication, semiconductor physics, MRI machines (which rely on quantum spin behaviors)… The inventions might be modern, but the science often loops back to Einstein’s breakthroughs. As one science writer put it, “the basis for today’s high technology” truly does rest on the quantum and relativistic principles Einstein advanced.
Einstein’s Legacy: A Century of Enlightenment and Beyond
It’s been over a century, and Einstein’s 1905 papers continue to resonate, inspire, and challenge us. They remind us that our intuitive picture of the world can be upended overnight by a bold new insight. Einstein was a master of the “thought experiment” – chasing beams of light in his imagination – but today we routinely confirm his ideas with real experiments.
In 2015, detectors observed gravitational waves, ripples in spacetime first predicted by Einstein’s relativity. In 2022, scientists demonstrated time dilation at mere millimeter scales using advanced clocks further merging quantum physics with gravity in ways Einstein could only dream of.
In 2022, the Nobel Prize honored experiments on quantum entanglement – a phenomenon Einstein highlighted in 1935 as a paradox – now the basis for quantum networks. It’s as if modern science is catching up to Einstein, finally putting empirical stamps on the revolutionary concepts he introduced.
Yet, Einstein’s story also shows the human side of science: a young man without a formal academic job, driven by “holy curiosity”, daring to question reality
He faced skepticism and resistance – his photon theory was so radical that even Planck and others took years to accept it
But Einstein had lit a fire that would not be extinguished. His Annus Mirabilis papers taught scientists how to think differently: to marry imagination with rigorous logic, to find simple postulates that resolve great paradoxes.
“Imagination is more important than knowledge. For knowledge is limited, whereas imagination encircles the world.”
Einstein later said.
His 1905 achievements encircled not just the world, but unlocked the subatomic realm and bent the fabric of spacetime.
Are there more “miracles” waiting in the wings? Physics today still has deep mysteries – dark matter, the unification of quantum mechanics with gravity – that echo the puzzles of Einstein’s day. Solving them may require an Einstein-like leap of intuition.
The legacy of 1905 is a challenge and an inspiration: it reminds us that even our most cherished assumptions about reality can change. What Einstein achieved in one miraculous year shows that with creativity and courage, we can transform our understanding of the universe.
As we marvel at GPS devices, quantum computers, or solar-powered rovers on Mars, we are beneficiaries of that miraculous year and the wisdom it continues to yield. The next time you tap your phone’s GPS or turn on a laser, take a moment to appreciate the century-old genius that made it possible – and imagine what paradigm shift might be next to change our perception of reality.